How Dark Matter Particles Can Confirm Cosmic Creation
One of the challenges lodged against the biblically predicted big bang creation model1 is the following query: Why haven’t scientists been able to detect the dark matter particles that are such a crucial component of standard big bang models? In fact, the models assert that 85% of the mass of the universe is composed of dark matter. How can we have confidence in the big bang model if we can’t find that 85%?
The answer from astronomers and physicists is that dark matter particles, regardless of how many such particles exist, by their very nature will be extraordinarily difficult to detect. Unlike ordinary matter, which is composed almost entirely of protons, neutrons, and electrons, dark matter either does not interact with light (photons) at all or interacts with light extremely weakly. Because of this very weak or nonexistent interaction, dark matter does not emit, absorb, or reflect light, which makes dark matter particles almost impossible to detect.
Detection and Measurements of Dark Matter
In spite of the fact that astronomers and physicists have thus far been unsuccessful in their attempts to detect dark matter particles, they nevertheless are convinced that dark matter exists. The reason why is that astronomers observe the effects of dark matter’s gravity on the behavior of galaxies and galaxy clusters. They note that the structure and long-term stability of galaxies can only be explained if galaxies are surrounded by huge haloes of matter that interact very weakly or not at all with light. Figure 1 shows the inferred dark matter halo that envelops our Milky Way Galaxy (MWG). Dark matter makes up about 90% of the MWG’s total mass.
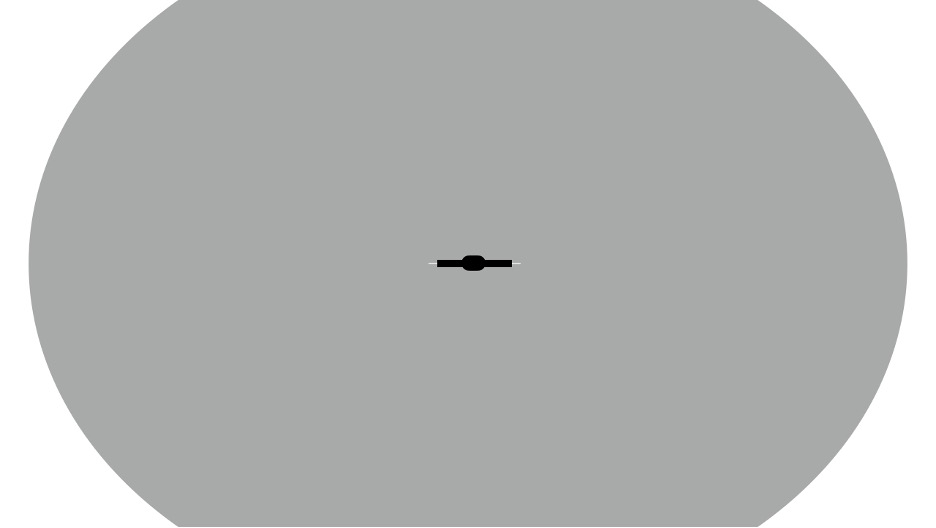
Figure 1: Milky Way Galaxy’s Dark Matter Halo and Stellar Disk
The central stellar bulge and the stellar disk are shown in black. The thin disk of gas appears in light gray. The much larger dark gray area depicts the dark matter halo. Credit: Hugh Ross
Astronomers also observe the effects of dark matter on maps of the cosmic microwave background radiation, also known as the radiation remaining from the cosmic creation event. These maps yield the most accurate measure of the total amount of dark matter in the universe. They reveal that dark matter comprises 85% of the total mass of the universe.2
Quest to Find Dark Matter Particles
Recent advances in cosmology and particle physics affirm that the cosmic creation model that best fits astronomical observations is fully compatible with the particle creation model that best fits the results of particle accelerator experiments. However, significantly more detailed and comprehensive particle and cosmic creation models would require the discovery and determination of the properties of dark matter particles. Consequently, for both astronomers and physicists the goal of discovering and measuring dark matter particles has become their “holy grail” quest.
The two leading hypothesized candidates for dark matter particles are high-mass particles called weakly interacting massive particles (WIMPs) and extremely low mass particles called axions. (As an aside, physicists displayed their marketing expertise by trademarking WIMPs so as to cash in on any manufacturers who desire to call any of their products a WIMP!)
The enormous mass difference between a WIMP and an axion implies that WIMPs will behave predominantly like discrete particles rather than like waves, whereas axions will behave predominantly like waves rather than like discrete particles. A team of astronomers led by Alfred Amruth has attempted to use this distinction to make headway in the quest to discover which particles comprise dark matter.3
According to Einstein’s theory of general relativity, the gravity of a massive object will bend light around itself. Ever since 1919, astronomers have routinely affirmed this prediction of general relativity by observing the bending of starlight passing close to the Sun’s disk during solar eclipses. Now, astronomers are taking advantage of this gravitational lensing to detect very distant galaxies and quasars directly behind a foreground galaxy or galaxy cluster. If the distant galaxy or quasar is lined up perfectly behind a foreground galaxy, its image will be smeared out into a circle—what astronomers call an Einstein ring. Figure 2 shows an example of a near perfectly aligned gravitational lensing of a distant galaxy into an Einstein ring image.
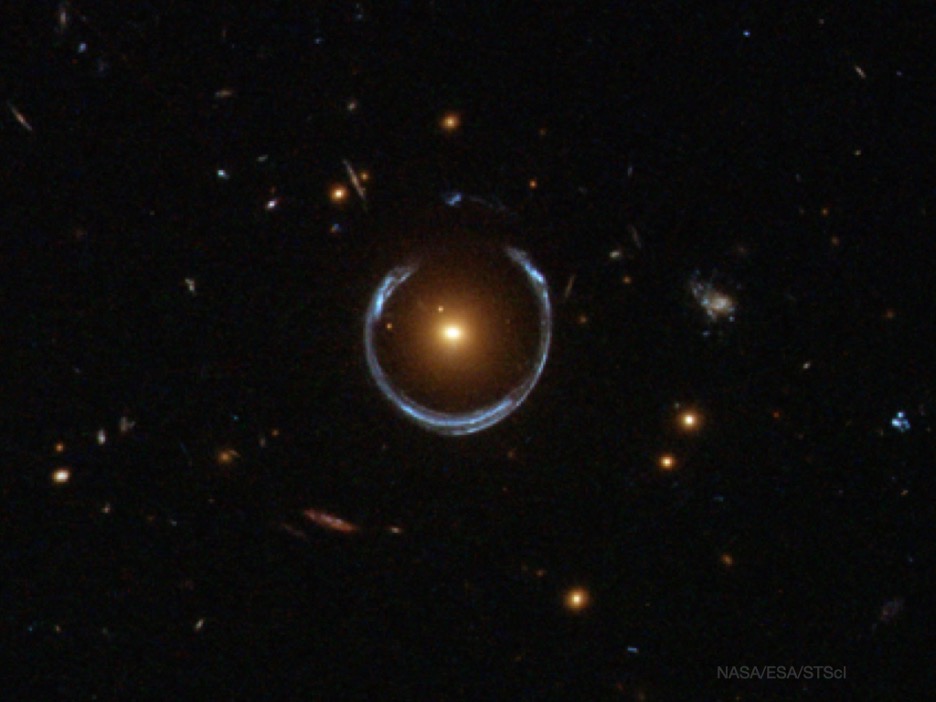
Figure 2: Gravitational Lensing of a Distant Galaxy into an Einstein Ring
Gravitational lens LRG 3-757. Credit: NASA/ESA/Hubble Space Telescope
Amruth and his colleagues developed several theoretical models to determine the different ways that gravitationally lensed images of distant galaxies/quasars would be distorted if the dark matter (that they know is responsible for most of the lensing phenomena) were composed predominantly of WIMPs or of axions. They then examined images of real gravitationally lensed galaxies to see whether WIMPs or axions best explained the observed features of the lensed galaxies/quasars.
The most definitive test in their sample was the gravitational lens around the foreground galaxy HS 0810+2554.4 However, since the alignment was not perfect, astronomers observed four bright spots instead of an Einstein ring around the foreground galaxy. The distant quasar gravitationally lensed by HS 0810+2554 manifests variable light intensity. Therefore, astronomers have observed multiple distinct gravitationally lensed images of the distant quasar (see figure 3).
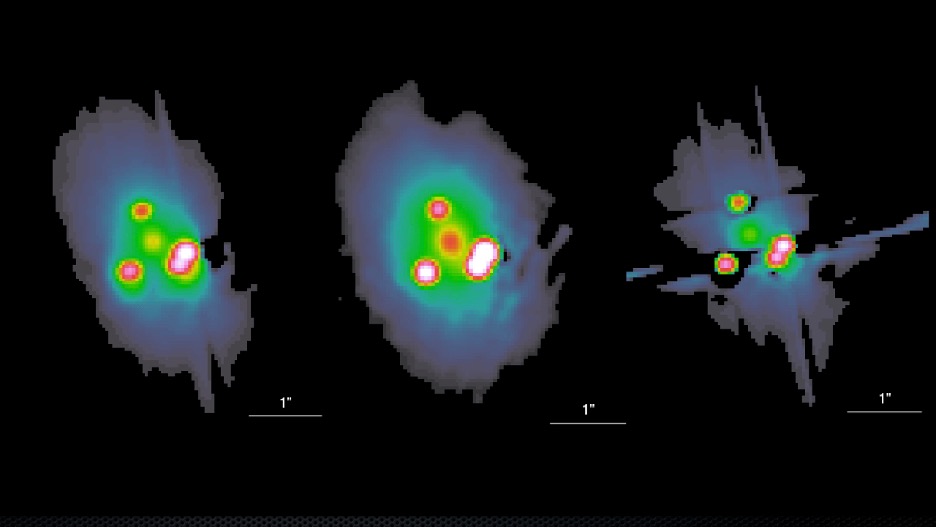
Figure 3: Three Observations at Different Times of a Quasar Gravitationally Lensed by HS 0810+2554
The scale bar to the bottom right of each image is 1 arcsecond. Credit: iweb.cfa.harvard.edu
In their entire sample of gravitationally lensed images of distant quasars, Amruth’s team noted that the images predicted by their WIMP models did not match the actual images. However, the images predicted by their axion models didaccurately reproduce the images, especially those of HS 0810+2554. In particular, they demonstrated that the brightness and position anomalies of the images gravitationally lensed by the foreground galaxy are well explained if the dark matter halo surrounding the galaxy is dominated by axions where the axion mass, to within a factor of ten, = 10-22 electron volts (for comparison, the mass of a proton = 928 million electron volts). Amruth and his colleagues also established that a dark matter halo surrounding HS 0810+2554 dominated by WIMPs fails to explain the observed features of the images gravitationally lensed by the galaxy. Consequently, the research team concluded that axions are a much more probable candidate than WIMPs for being the universe’s predominant dark matter particle.
This research is the strongest evidence to date favoring axions as the dominant dark matter particle. However, it is not the only evidence favoring axions. The axion and WIMP dark matter particle models predict different kinds and populations of dwarf galaxies in the universe. The WIMP models predict that dark matter haloes will dramatically increase in number toward lower masses, with numbers dropping to zero at a dark matter halo mass of 1,000 solar masses. The axion models predict that the numbers of dark matter haloes will be increasingly suppressed below masses of about a billion times the Sun’s mass, assuming an axion mass of 10-22 electron volts.5 The mass where suppression of dark matter haloes occurs is roughly proportional to the axion mass.
Counts of the number of low mass dwarf galaxies in the Local Group favors the axion models over the WIMP models. Likewise, the observed abundance of low luminosity galaxies at high redshifts (large cosmological distances) favors axion models and strongly disfavors WIMP models.6 The axion models also explain why dwarf galaxies do not exhibit cusps,7 a feature that WIMP models can explain only by appealing to star formation feedback. Axion models, unlike WIMP models, can explain the stellar velocity dispersion of DF44, an ultra-diffuse dwarf galaxy.8
Astronomers now possess the telescope power to observe the structure of the universe on the largest size scales. Observations conducted to date reveal a large-scale structure of the universe that fits the model holding that the universe’s dark matter is composed predominantly of axions.9 Astronomers have also observed white dwarf stars where the white dwarfs are cooling at faster rates than what thermodynamics alone would predict. The additional cooling could be explained if large numbers of axions exist in the white dwarf stars and if some of those axions are escaping or decaying.10
Amruth and his team concluded their paper by noting that major gravitational lens surveys are underway. The database of well-observed gravitationally lensed images of distant quasars will exponentially increase within the next several years. Such a database could not only definitively establish axions as the predominant dark matter particle but also provide an accurate measure of the axion mass. Meanwhile, laboratory experiments to detect axions are underway and more sensitive experiments are being proposed and developed.11
Philosophical Implications
The research achieved by Amruth and his teammates carries significant implications. It dispels the claim that scientists will never detect dark matter particles and removes any reasonable doubt of dark matter’s existence. It also provides yet more scientific evidence in support of the biblically predicted big bang creation model. Already, it has provided a more detailed and comprehensive model for the origin and history of the universe and for the origin and history of the universe’s fundamental particles.
This work shows the research pathway for developing increasingly detailed and comprehensive cosmic and particle creation models. We can all look forward to the results. Once again, scientific advance affirms Psalm 19:1: “The heavens declare the glory of God.”
Check out more from Reasons to Believe @Reasons.org
Endnotes
- Hugh Ross, “What Does the Bible Say about the Big Bang?” Today’s New Reason to Believe (blog), Reasons to Believe, February 6, 2023.
- G. Hinshaw et al., “Nine-Year Wilkinson Microwave Anisotropy Probe (WMAP) Observations: Cosmological Parameter Results,” Astrophysical Journal Supplement 208, no. 2 (October 2013): id. 19, 5–11, doi:10.1088/0067-0049/208/2/19; P. A. R. Ade et al., Planck Collaboration, “Planck 2015 Results. XIII. Cosmological Parameters,”Astronomy & Astrophysics 594 (October 2016): id. A13, 1, doi:10.1051/0004-6361/201525830; David N. Spergel, Raphael Flauger, and Renée Hložek, “Planck Data Reconsidered,” Physical Review D 91 (January 27, 2015): id. 023518, 1, doi:10.1103/PhysRevD.91.023518.
- Alfred Amruth et al., “Einstein Rings Modulated by Wavelike Dark Matter from Anomalies in Gravitationally Lensed Images,” Nature Astronomy (April 20, 2023), doi:10.1038/s41550-023-01943-9.
- Neal Jackson et al., “Observations of Radio-Quiet Quasars at 10-mas Resolution by Use of Gravitational Lensing,” Monthly Notices of the Royal Astronomical Society 454, no. 1 (November 2015): 287–298, doi:10.1093/mnras/stv1982.
- Lam Hui et al., “Ultralight Scalars as Cosmological Dark Matter,” Physical Review D 95, no. 4 (February 15, 2017): id. 043541, doi:10.1103/PhysRevD.95.043541.
- Enoch Leung et al., “Magnification Bias of Distant Galaxies in the Hubble Frontier Fields: Testing Wave Versus Particle Dark Matter Predictions,” Astrophysical Journal 862, no. 2 (August 1, 2018): id. 156, doi:10.3847/1538-4357/aacdad.
- Tom Broadhurst et al., “Ghostly Galaxies as Solitons of Bose-Einstein Dark Matter,” Physical Review D 101, no. 8 (April 15, 2020): id. 083012, doi:10.1103/PhysRevD.101.083012.
- Alvaro Pozo et al., “Detection of a Universal Core-Halo Transition in Dwarf Galaxies as Predicted by Bose-Einstein Dark Matter,” (December 17, 2021), preprint arXiv:2010.10337.
- Hsi-Yu Schive, Tzihong Chiueh, and Tom Broadhurst, “Cosmic Structure as the Quantum Interference of a Coherent Dark Wave,” Nature Physics 10 (July 2014): 496–499, doi:10.1038/nphys2996.
- J. Isern et al., “Axions and the Luminosity Function of White Dwarfs: The Thin and Thick Discs, and the Halo,” Monthly Notices of the Royal Astronomical Society 478, no. 2 (August 2018): 2569–2575, doi:10.1093/mnras/sty1162; J. Isern et al., “Axions and the Pulsation Periods of Variable White Dwarfs Revisited,” Astronomy & Astrophysics 512 (March 2010): id. A86, doi:10.1051/0004-6361/200913716; J. Isern et al., “Axions and the Cooling of White Dwarf Stars,” Astrophysical Journal Letters 682, no. 2 (August 1, 2008): L109–L112, doi:10.1086/591042; Jordi Isern, “White Dwarfs as Advanced Physics Laboratories. The Axion Case,” in White Dwarfs as Probes of Fundamental Physics: Tracers of Planetary, Stellar, and Galactic Evolution, edited by Martin A. Barstow et al., Proceedings of the International Astronomical Union 357 (October 9, 2020): 138–153, doi:10.1017/S1743921320000873.
- Yannis K. Semertzidis and Sungwoo Youn, “Axion Dark Matter: How to See It?” Science Advances 8, no. 8 (February 23, 2022): id. abm9928, doi:10.1126/sciadv.abm9928.