Cosmic Dawn Evidence Bolsters Case for Creation
During this first year of its operation, the James Webb Space Telescope (JWST) has made several remarkable discoveries. I’ve reported on these findings in my posts, “James Webb Space Telescope: Initial Revelations” and “Solving Star Formation Mysteries Affirms Big Bang Creation.” In just the past few days, the JWST has helped a team of 36 astronomers to make an even more significant discovery about the early history of the universe.1 This breakthrough affirms yet another prediction of the big bang creation model.2
To help readers who are not astrophysicists to appreciate this discovery, let me provide some background.
Cosmic Dawn
Astronomers refer to one of the most crucially important epochs in the universe’s history as the cosmic dawn. It’s the time when the first stars form and their nuclear furnaces ignite so that they shine. In this early moment, the universe transitions from ubiquitous darkness to pervasive brightness.
The JWST’s primary long-term mission is to explore and determine the nature of this cosmic dawn. Specifically, astronomers want to know how massive and numerous these first-born stars were and how they affected the formation of the universe’s first galaxies and black holes. Different big bang creation models predict different populations and mass ranges of such stars (called Population III stars).
Challenge of Seeing the Very First Stars
Astronomers have long known that not all stars form at the same rate. The greater the mass and density of a gas cloud, the faster that cloud will collapse and produce a protostar (young star that is still gathering and compressing the mass needed to ignite nuclear fusion). The greater the mass of the protostar, the faster the nuclear fusion in its core (the fusion of hydrogen into helium) will ignite to make it a stable, bright, shining star.
The shortest time in which a gas cloud can produce a protostar is about 1 million years.3 The longest it may take is about 10 million years. Table 1 lists the time required for protostars of different masses to transition from first becoming protostars to igniting the nuclear fusion of hydrogen into helium in their cores.4 Knowing these times helps astronomers gain a more accurate picture of the universe’s early history.
Table 1: Time Durations for Protostars to Become Nuclear-Burning Stars
Star mass at nuclear ignition time Time until nuclear-burning ignition
100 solar masses | <3,000 years |
15 solar masses | 60,000 years |
5 solar masses | 575,000 years |
1 solar mass | 50,000,000 years |
0.3 solar masses | 300,000,000 years |
0.1 solar masses | 1,000,000,000 years |
Table 2 lists the time required for stars to complete their nuclear burning cycle from start to finish and become supernovae. In a supernova event, a star blasts most of its nuclear-burning products into interstellar space. Stars that end their nuclear burning with masses more than 1.4 times the Sun’s mass will undergo a supernova eruption.5 Only stars that begin their nuclear burning with a mass greater than or equal to about 10 times the Sun’s mass will end their nuclear burning with this much mass. The duration of a star’s nuclear burning cycle is inversely proportional to the 2.5 power (~M-2.5) to its mass at the time nuclear burning ignites.
Table 2: Times for Stars to Complete Their Nuclear Burning and Become Supernovae
Star mass at nuclear ignition time Time from nuclear ignition until supernova
200 solar masses | 18,000 years |
100 solar masses | 100,000 years |
40 solar masses | 1,000,000 years |
10 solar masses | 35,000,000 years |
As Tables 1 and 2 show, the most massive Population III stars in the universe burn through their nuclear fuel and explode as supernovae in 18,000 years or less (table 2) before the least massive Population III stars even begin their nuclear burning in 1 billion years (table 1). In fact, the most massive Population III stars will go through their entire nuclear-burning cycle before the least massive ones completely form as protostars from within the universe’s first gas clouds.
The dramatic difference in star formation time means that all the universe’s low-mass Population III stars will inevitably be polluted by the ashes from the high-mass Population III stars well before nuclear burning even ignites in these low-mass stars. This difference means that only the highest-mass Population III stars will be free of chemical pollution from supernova eruption events.
Pristine Population III Stars
All big bang models predict that the universe begins with just one element in the periodic table: hydrogen. At the cosmic creation event, the universe is nearly infinitely hot. As the universe expands, it cools. One of the strongest verifications of big bang cosmology is the 12 billion-year cooling curve astronomers have observed and measured. It perfectly matches what the big bang models predict.6
During the first few minutes after the cosmic beginning, the universe spends about 20 seconds in the 14–150 billion Kelvin temperature window, where nuclear fusion is able to take place. Within these 20 seconds, about 24% of the universe’s primordial hydrogen is fused into helium, along with a trace amount of lithium.
Big bang models thus predict that the very first stars to form will be composed of only these three elements: hydrogen, helium, and lithium. All other elements will arise from the nuclear furnaces of stars, from supernovae, and from neutron star mergers.7
The challenge for astronomers has been that only the most massive Population III stars will have avoided being polluted by the ashes of supernovae. These extremely massive stars shine brightly for only a few tens of thousands of years. This rapid burn-up means that astronomers will only be able to image them at look-back times corresponding to the very early cosmic epoch when conditions first permit star formation to occur: the cosmic dawn. In other words, astronomers will need to search for these stars at distances of about 13.6 billion light-years—close to the earliest moments of the universe’s 13.8 billion-year history.
Not even the JWST possesses the power to image individual stars at distances of 13.6 billion light-years. The best that astronomers can hope for is to image a large dense cluster of stars at this distance. However, given how rapidly the most massive stars form, burn, and explode, such a star cluster is bound to be at least partly polluted by the earliest-forming stars, making the detection of stars comprised of only hydrogen, helium, and a trace amount of lithium extremely difficult.
Nearly Pristine Population III Stars
The fact that stars composed of only hydrogen, helium, and a trace amount of lithium are observationally out of reach does not mean, however, that astronomers have no hope of establishing that such stars existed in the early universe. This important prediction of big bang cosmology can be verified by the discovery of low-mass Population III stars that have been polluted (infused with elements) exclusively by the residue of stars that started their nuclear burning with only hydrogen, helium, lithium, and no other elements.
These low-mass Population III stars remain bright for billions of years. Therefore, astronomers need not look back as far as 13.6 billion years (or the equivalent distance) to see them.
To date, astronomers have discovered some low-mass Population III stars in the outer halo of our Milky Way Galaxy (MWG).8 As described in my book, Designed to the Core, our MWG is extraordinary in that its outer halo has an extremely low density of stars,9 with an especially sharp drop-off in density beyond 90,000 light-years from the MWG center. Astronomers have also determined that these outskirt stars have remained dynamically undisturbed for the past 11 billion years.10 Because of the unique features of the MWG’s outer halo, a few of the universe’s Population III stars still exist there and have not been polluted by later-forming stars (called Population II, which form entirely from the ashes of exploded Population III stars, and Population I stars, which form predominantly from the ashes of exploded Population II stars). They’ve been polluted only by the ashes of other Population III stars.
The spectral signatures (measures of atmospheric composition) of these stars show that they possess extremely low quantities of elements heavier than lithium, quantities lower by orders of magnitude (factors of hundreds of times) than the least polluted Population II stars. Thus, there is no mistaking that they are Population III stars.
Breakthrough: Metal-Free Population III Stars
Astronomers would still hope to find direct evidence of “metal-free” Population III stars, stars manifesting huge amounts of hydrogen and helium and no measurable quantity of elements heavier than lithium (in astronomers’ terms,“metals”).
Thanks to the JWST, such direct evidence of metal-free Population III stars may now be in hand. A mission known as JADES (JWST Advanced Deep Extragalactic Survey) is a quest by dozens of astronomers from all over the world to image the cosmic dawn in far greater detail and depth than the Hubble Space Telescope made possible.
In a paper already submitted to and still undergoing peer review at Astronomy & Astrophysics, 36 astronomers led by Roberto Maiolino used the JWST to study, spectroscopically, the halo of the galaxy GN-z11 (see figure 1). GN-z11 is an exceptionally luminous galaxy, ranked as the brightest known galaxy in the first half billion years of cosmic history.11 GN-z11 has a light-travel distance of 13.35 billion light-years, which tells us that we are seeing it as it was just 440 million years after the big bang origin event.
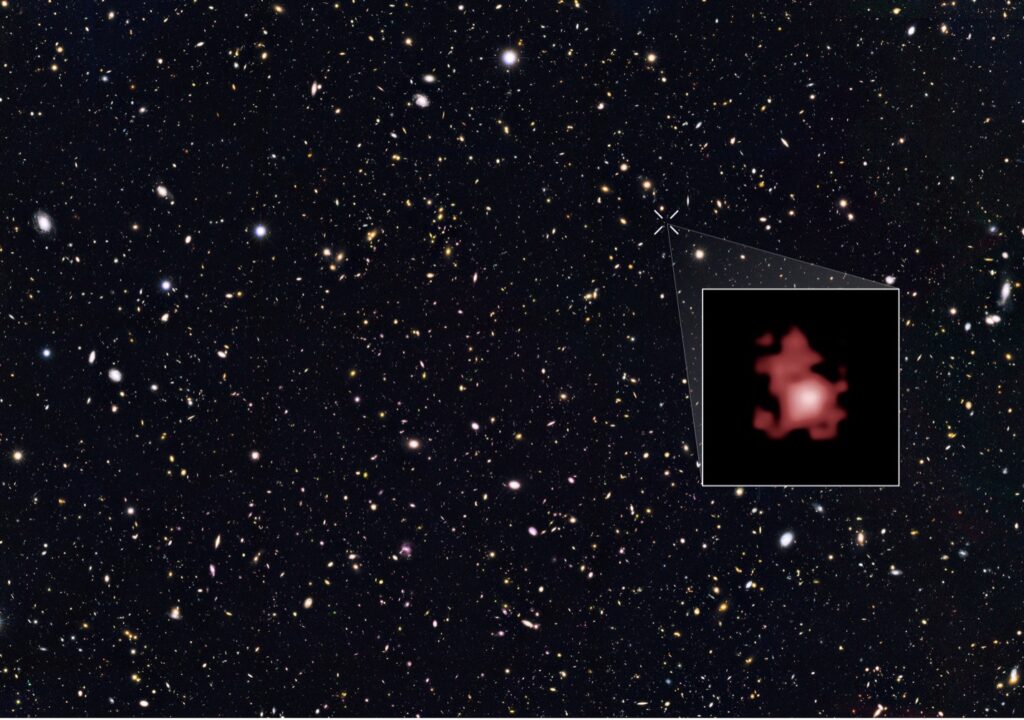
Figure 1: Hubble Space Telescope Image of GN-z11
Credit: NASA/ESA
GN-z11 has 1% of the mass and about 5% of the size of the MWG, and yet it’s one of the largest galaxies in the very early universe. GN-z11’s total stellar mass is about a billion times the Sun’s mass and its stars have an average age of only 40 million years (young, in astronomical terms).12
Maiolino and his colleagues detected the HeIIl1640 emission line in the halo of GN-z11. They then went on to show that the unusual width of this spectral line, as well as the absence of metal spectral lines, is best explained by the presence of extremely massive Population III stars. These stars—with masses ranging from 100 to 500 times (or greater) the mass of the Sun—would have effectively photoionized all the gas in GN-z11’s halo. The team’s data simultaneously ruled out Population II stars or GN-z11’s active galactic nucleus as possible source(s) of the observed photoionization.
The existence of metal-free stars in GN-z11 with masses in the 100–500+ range, measured by Maiolino and his colleagues, helps explain GN-z11’s exceptionally high mass and size. This discovery yields the most direct and definitive evidence to date for the existence of the mass range of Population III stars predicted by virtually all big bang models. It provides the clearest picture to date of the cosmic dawn.
A Bright Way Forward
It’s a momentous discovery, but it must be confirmed and more solidly established. As Maiolino’s team notes, astronomers will need the JWST to take deeper images of GN-z11 and other distant galaxies like it. (In an upcoming post, I’ll describe a new tool that astronomers plan to use to explore the cosmic dawn—a low-cost telescope to be placed on the Moon’s far side with the capacity to detect wavelengths of light never before seen by astronomers.) Already, however, this research has shown the astronomical community and the wider world the value of the JWST in providing fresh insights into the cosmic dawn.
As astronomers gain further understanding of the numbers and mass distribution of the earliest stars, they’ll be able to build better models for the formation and development of the universe’s first galaxies. This bottom-up approach will be complemented, potentially, by a top-down strategy, which entails using the JWST to gather data on the universe’s first galaxies and how they formed.
One major benefit of this latest discovery is this: as astronomers learn more about the cosmic dawn, their insights will reveal which of several big bang creation models provides the most accurate description of the origin and history of the universe. I’m confident that a more detailed and precisely defined big bang model will yield yet more evidence that what the Bible declared thousands of years ago about the origin and the history of the universe is, in fact, true. Thus, it will strengthen the case for the divine inspiration and accuracy of the Bible, giving people around the world more reasons to believe in the One who created the universe and inspired the Scriptures.
Check out more from Reasons to Believe @Reasons.org
Endnotes
- Roberto Maiolino et al., “JADES: Possible Population III Signatures at z = 10.6 in the Halo of GN-z11,” arXiv:2306.00953v2 (June 6, 2023), submitted to Astronomy & Astrophysics.
- Hugh Ross, “What Does the Bible Say about the Big Bang?” Today’s New Reason to Believe (blog), Reasons to Believe, February 6, 2023.
- Richard B. Larson, “The Physics of Star Formation,” Reports on Progress in Physics 66 (September 10, 2003): 1651–1697, doi:10.1088/0034-4885/66/r03.
- Masanobu Kunitomo et al, “Revisiting the Pre-Main-Sequence Evolution of Stars: I. Importance of Accretion Efficiency and Deuterium Abundance,” Astronomy & Astrophysics 599 (March 2017): id. A49, doi:10.1051/0004-6361/201628260; I.-Juliana Sackmann, Arnold I. Boothroyd, and Kathleen E. Kraemer, “Our Sun. III. Present and Future,” Astrophysical Journal 418 (November 20, 1993): 457–468, doi:10.1086/173407; Rudolf Kippenhahn and Alfred Weigert, Stellar Structure and Evolution (Berlin: Springer Verlag, 1994), 266–270.
- Eduardo Bravo et al., “Chandrasekhar-Mass White Dwarfs Are the Progenitors of a Small Fraction of Type Ia Supernovae According to Nucleosynthesis Constraints,” Monthly Notices of the Royal Astronomical Society Letters 517, no. 1 (November 2022): L31–L35, doi:10.1093/mnrasl/slac103; Paolo A. Mazzali et al., “A Common Explosion Mechanism for Type Ia Supernovae,” Science 315, no. 5813 (February 9, 2007): 825–828, doi:10.1126/science.1136259.
- Hugh Ross, “Taking the Big Bang’s Temperature,” Today’s New Reason to Believe (blog), Reasons to Believe, April 4, 2022.
- Hugh Ross, “Neutron Star Merger Explains Why We’re Here (Expanded Version),” Today’s New Reason to Believe (blog), Reasons to Believe, November 3, 2017.
- Hugh Ross, “Big Bang Implications of Detecting the Universe’s First Stars,” Today’s New Reason to Believe (blog), Reasons to Believe, November 14, 2022; Hugh Ross, “J0023+0307, a Pristine Firstborn Star?” Today’s New Reason to Believe (blog), Reasons to Believe (April 9, 2018); Hugh Ross, “Pursuing the Firstborn Stars and a Better Cosmic Creation Model,” Today’s New Reason to Believe (blog), Reasons to Believe (July 15, 2019).
- Hugh Ross, Designed to the Core (Covina, CA: RTB Press, 2022), 90–92.
- Hugh Ross, “Have Galactic Archaeologists Found Evidence for Design?” Today’s New Reason to Believe (blog), Reasons to Believe, June 6, 2022.
- P. A. Oesch et al., “A Remarkably Luminous Galaxy at Z = 11.1 Measured with Hubble Space Telescope Grism Spectroscopy,” Astrophysical Journal 819, no. 2 (March 8, 2016): id. 129, doi:10.3847/0004-637X/819/2/129.
- Oeasch et al., “A Remarkably Luminous Galaxy,” p. 7.