LuSEE: Boldly Going Where No One Has Gone Before
As astronomers explore the distant regions of the universe, they look for electromagnetic radiation, energy that spreads outward as it travels through the cosmos. Tracking this radiation allows astronomers to study how the universe originated and developed throughout its history.
Electromagnetic radiation comes in a range of wavelengths that make up the electromagnetic spectrum. The range includes AM radio waves at the lowest energy/longest wavelength end of the spectrum and gamma-rays at the highest energy/shortest wavelength end. Astronomers have observed every part of the electromagnetic spectrum except one: radio frequencies below 30 megahertz, corresponding to wavelengths longer than 10 meters.
Now, thanks to a much-anticipated mission scheduled for deployment in 2025 or early 2026, astronomers are about to explore the final frontier.1 I expect that the knowledge gained from this exploration will yield new evidence for the intentional design of the cosmos for our existence.
Impenetrable Barrier: Earth’s Ionosphere
One reason astronomers have been unable to explore the universe at wavelengths longer than 10 meters has to do with the effects of Earth’s ionosphere, the region where Earth’s atmosphere meets interplanetary space. Earth’s ionosphere extends from 48 to 965 kilometers (30–600 miles) above sea level. It’s where ultraviolet and x-ray radiation from the Sun ionizes (strips away the electrons of) atoms and molecules.
This ionization in Earth’s upper atmosphere generates a huge amount of radio “noise” at wavelengths longer than 3 meters. All that noise limits astronomers’ capability to glean meaningful information about our galaxy, other galaxies, and the distant universe at those wavelengths.2 Nevertheless, because of the importance of this wavelength range, astronomers have dedicated five instruments to making observations at wavelengths longer than 3 meters: (1) the Square Kilometer Array (SKA), (2) the Low Frequency Array (LOFAR), (3) Large Array Low-Frequency Sky Survey (VLSS), (4) the Galactic and Extragalactic All-Sky MWA Survey (GLEAM), and (5) the Eighth Cambridge (8C) Survey.
The longest wavelength yet observed by the first four instruments has been 4.2 meters. Astronomers used the fifth instrument to observe radio sources at 7.9 meters; however, the quality of their data was marginal.3
At wavelengths longer than 10 meters, Earth’s ionosphere is almost entirely opaque. Consequently, ground-based telescopes have been unable to detect radio sources at those wavelengths coming from beyond the solar system.
Impenetrable Barrier: Radio Transmissions
At wavelengths longer than 3 meters, the electromagnetic spectrum is saturated by AM and FM radio station broadcasts, short-wave radio transmissions, ham radio transmissions, VHF television broadcasts, and military over-the-horizon radar.4 Decades ago, there were a few locations on Earth where one could avoid such radio transmissions. Not anymore.
The number of radio, radar, and television transmitters has multiplied exponentially. At wavelengths longer than 3 meters, Earth’s ionosphere reflects radio signals with an efficiency that is proportional to the wavelength. The radio noise just from AM radio stations is huge. For example, there are 220 AM radio stations broadcasting at 50,000 watts in the United States alone. For comparison, the extragalactic radio sources astronomers desire to detect at wavelengths longer than 10 meters are weaker than 10-26 watts per square meter per hertz. Thus, nowhere on Earth can astronomers’ instruments detect radio emissions from stars, galaxies, and nebulae at wavelengths longer than 10 meters.
Escaping the Barriers
With Earth’s surface ruled out for very-long-wavelength radio astronomy, astronomers have considered Earth-orbiting telescopes. However, radio transmission that leaks through Earth’s ionosphere creates a major interference.
Another problem is that the longer the wavelength astronomers observe, the greater the uncertainty in determining the positions and sizes of the radio sources. To gain sufficient resolution to determine the sizes and positions of radiation emissions at such wavelengths, astronomers must construct interferometers.
In radio interferometry, astronomers combine the radiation signals they receive from multiple telescopes that are spatially separated from one another to create a high-resolution image of the astronomical source. However, for radio interferometry to deliver a reliable image, astronomers must continuously know the precise positions of each telescope in the array and the timing of incoming waves to each telescope. This requirement is attainable only when all the telescopes are mounted on a single fixed, solid body like Earth where the position of each telescope is accurately known and each telescope possesses a calibrated high-precision clock. An example of this arrangement is the Very Long Baseline Array, a set of 10 radio telescopes, each equipped with a sophisticated hydrogen maser atomic clock, stretching from Hawaii to the US Virgin Islands. It is much more challenging, by far, when the radio telescopes are in separate orbits about Earth.
Moon’s Far Side: The Last Refuge
For long-wavelength radio astronomy, the far side of the Moon (see figure 1) is the only unspoiled detection location in the solar system. It’s the only place where Earth’s intense radio transmissions and noisy ionosphere are permanently blocked out. It’s the only place where astronomical observation at wavelengths longer than 10 meters is even possible. For observations in the 3 to 10-meter range, it remains the only place where astronomers can detect faint signals from far distant galaxies and quasars.
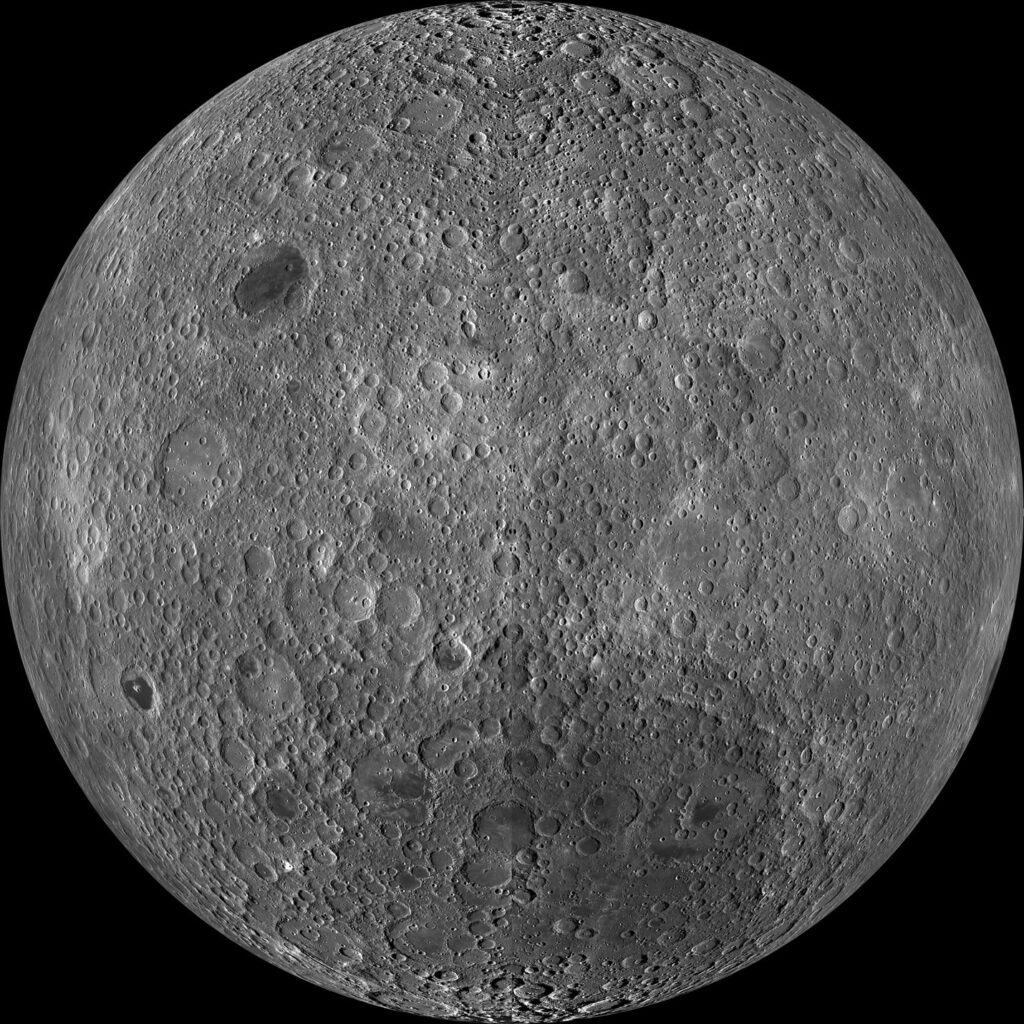
Figure 1: Moon’s Far Side
Credit: NASA
The Moon’s far side is also the only unspoiled haven for broadband astronomical observations in the 1-centimeter to 3-meter wavelength range. In that range, radio astronomy has been squeezed into a few protected narrow bands. This loss of detection sensitivity is not trivial. The Moon’s far side is the one place where the detection sensitivity that broadband observations make possible can potentially be recovered.
Going Where No One Has Gone Before
While twenty-first-century technology has seriously disrupted Earth-based radio astronomy, it also holds promise. I did my PhD research long ago, during the Apollo program, and I remember talking to my professors back then about the benefits of putting radio telescopes on the back side of the Moon. They laughed. The technology didn’t exist at that time to construct radio telescopes anywhere on the Moon, much less on the back side.
We’re no longer so limited. Today, the technology does exist to place radio telescopes on the Moon—even on its far side—and at relatively low cost. NASA and the US Department of Energy have developed a robotic telescope that will attempt first-of-its-kind measurements.
LuSEE–Night Instrument
This new instrument, the Lunar Surface Electromagnetics Experiment—Night (LuSEE–Night) consists of four monopole antennae arranged in a cross-configuration and mounted on top of a rotating platform (see figure 2). The plan is to place it in the CS-13 region of the Moon’s far side via a Commercial Lunar Payload Services (CLPS) mission scheduled, as mentioned above, for late 2025 or early 2026. Since it will be fully robotic, the mission will include all the technology needed to deliver the LuSEE-Night instrument to the Moon’s far side, set it up, and make it fully operational. No astronauts will be needed.
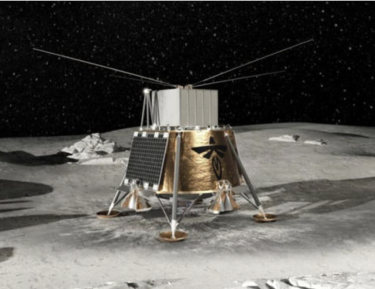
Figure 2: LuSEE–Night Instrument
Credit: NASA/DOE
The LuSEE–Night landing site is a 100-meter ellipse centered on the lunar far side near the antimeridian. The chosen site is near the antipode (the location most opposite to Earth) so as to minimize terrestrial radio frequency interference. There is not a total blockout of radio interference from Earth since at the lowest frequencies some noise will likely be refracted by the tenuous lunar ionosphere.5 The landing site was also chosen for its relative flatness and low horizon.
LuSEE–Night’s four monopole antennae will observe the radio sky and feed a full-bandwidth spectrometer operating in the frequency range of 0.1 to 50 megahertz, corresponding to wavelengths ranging from 6 meters to 3,000 meters. If it works as planned, it will give astronomers their first-ever glimpse at objects beyond our solar system at wavelengths longer than 7.9 meters.
Observations will be conducted during the 14-day, or 340-hour, “lunar night” when the Moon’s far side is pointed away from the Sun so that any interference from solar radiation will be blocked out. The time researchers have chosen for observations will also minimize radio interference from Jupiter and Saturn. LuSEE–Night will be powered up at the beginning of the lunar night and will be permanently powered down at the end of the lunar night. This timing will eliminate the risk of interference from electromagnetic emissions generated by the LuSee spacecraft, which could threaten the integrity of the observations.
LuSEE–Night’s antennae patterns and observations will be calibrated by known 10-second-duration signals from a CLPS lunar orbiter. This orbiter will send timed signals to LuSEE-Night throughout its observing run.
Discovering What the Heavens Reveal
LuSEE–Night’s scientific mission is to study the astronomical “Dark Ages.” The Dark Ages is the one unexplored portion of cosmic history—the era between the last scattering of the cosmic microwave background radiation (CMBR) and the formation of the universe’s first stars. The last scattering of the CMBR occurred 380,000 years after the cosmic creation event, and the universe’s first stars formed 200–300 million years after that event. Only a cold, non-luminous mixture of hydrogen and helium gases existed during that era, as far as we know.
Radio waves at wavelengths longer than 10 meters represent the only signals astronomers can measure from the Dark Ages. Therefore, LuSEE provides an opportunity for astronomers to learn how some of the universe’s first nonluminous matter condensed to form the universe’s first stars.
Astronomers anticipate that their investigation of the Dark Ages will affirm which of several LCDM (lambda cold dark matter) inflationary big bang models most accurately describes the origin and history of the universe. LCDM models are those in which most of the universe is comprised of dark energy and most of the remainder of the universe is composed of cold dark matter. Part of exploring the Dark Ages includes the cosmic dawn, the appearance of the universe’s first stars. As described in last week’s article (“Cosmic Dawn Bolsters Case for Creation”), the James Webb Space Telescope will be exploring events that occurred from the cosmic dawn onward to the universe’s first galaxies and first galaxy clusters. The LuSEE–Night and, eventually, much larger radio arrays on the Moon’s far side, could allow us to explore the events leading up to the cosmic dawn. Together these two observing programs have potential to penetrate astronomy’s last frontier, taking us to previously hidden moments in cosmic history.
As Anže Slosar, the Department of Energy science lead and LuSEE–Night Collaboration spokesperson comments, “Every time we have opened a new frequency window in cosmology, we have unlocked new discoveries about the history of the universe and our place within it.”6 I expect the missions to reveal new cosmic design features and more insights into how the universe was purposefully and precisely prepared for the entry of humanity. We can all look forward to the revelation of even more of God’s glory and goodness (Psalm 19:1–4; Psalm 50:6, Psalm 96:7).
Check out more from Reasons to Believe @Reasons.org
Endnotes
- Stuart D. Bale et al., “LuSee ‘Night’: The Lunar Surface Electromagnetics Experiment,” arXiv:2301.10345 (January 24, 2023), doi:10.48550/arXiv.2301.10345.
- F. de Gasperin et al, “The Effect of the Ionosphere on Ultra-Low-Frequency Radio-Interferometric Observations,” Astronomy & Astrophysics 615 (July 2018): id. A179, doi:10.1051/0004-6361/201833012.
- Mark Lacy, Steve Rawlings, and P. J. Warner, “A Complete Sample of Radio Sources in the North Ecliptic Cap, Selected at 38 MHz—I. The Radio Data,” Monthly Notices of the Royal Astronomical Society 256, issue 3 (June 1992): 404–424, doi:10.1093/mnras/256.3.404.
- M. L. Kaiser et al., “WIND/WAVES Observations of Man-Made Radio Transmissions,” Geophysical Research Letters 23, no. 10 (May 15, 1996): 1287–1290, doi:10.1029/95GL03665.
- Neil Bassett, et al., “Characterizing the Radio Quiet Region behind the Lunar Farside for Low Radio Frequency Experiments,” Advances in Space Research, 66, no. 6 (September 15, 2020): 1265, doi:10.1016/j.asr.2020.05.050.
- Anže Slosar, quoted in US Department of Energy: Office of Science, “Department of Energy and NASA Join Forces on Innovative Lunar Experiment,” March 7, 2023.