The Genetic Code: Optimized for Resource Conservation
“Waste not, want not.”
Maria Edgeworth, The Parent’s Assistant, 1796
It is rare to hear this bit of sound advice today, given we live in a throwaway society. But growing up, I often witnessed my parents living by this adage.
My mom’s parents lived through the Great Depression so she learned never to throw things away that might be useful later. My father’s family lost everything they had as a result of the Partition of India (when India won its independence from Great Britain in 1947). As a result of that experience, my father became extremely frugal.
Conserving goods and energy makes sense. If we don’t needlessly waste things during times of abundance, then we are less likely to be without them when things are sparse.
As it turns out, researchers have discovered that the design of the genetic code evinces a biochemical version of the “waste not, want not” principle.1 Investigators from Israel have learned that the genetic code is optimized for resource conservation. This optimization is vital, contributing to life’s ability to persist during times when carbon- and nitrogen-containing resources in the environment become depleted.
The Importance of Resource Conservation
Carbon and nitrogen hold immense value for living organisms, serving as two of life’s key raw materials. In fact, ecologists regard the carbon and nitrogen levels in an ecosystem as limiting factors. In other words, the amount of available carbon and nitrogen in the environment controls an ecosystem’s biomass (amount of living matter). When the levels of nutrients containing these two elements are low, the ecosystem can’t sustain the number of organisms that it can when the levels of carbon- and nitrogen-containing nutrients are high.
Life-forms use carbon and nitrogen atoms to assemble the building block materials that, in turn, are used to construct important biomolecules such as proteins, the nucleic acids (DNA and RNA), and cell membrane components such as phospholipids. Additionally, organisms use carbon-containing compounds as a source of energy to power their operations.
Not all biomolecules are created equal. Some require more carbon and nitrogen atoms to assemble than others. Consider proteins. These biomolecules are built from amino acids that have been linked together in a chain-like manner by the cell’s biosynthetic machinery. As a general rule, the cell’s machinery uses twenty chemically and physically distinct amino acids to build proteins. The assembly of each of these amino acids requires differing amounts of carbon and nitrogen atoms, with some necessitating more carbon atoms or, conversely, more nitrogen atoms than others.
Biochemists have learned that the composition of biomolecules is influenced by nutrient availability. Organisms that live in low-carbon environments tend to have proteins that are predominantly made up of amino acids that are relatively rich in nitrogen. However, organisms that reside in a low-nitrogen environment possess proteins that are relatively low in nitrogen-rich amino acids.
Biochemists have also observed that the composition of an organism’s genome reflects the availability of nutrients in the environment. The DNA that makes up an organism’s genome consists of linear chains of nucleotides. The cell’s biosynthetic apparatus assembles DNA from four nucleotides: adenosine, guanosine, cytidine, and thymidine (abbreviated A, G, C, and T, respectively).
Organisms that live in low-carbon environments have genomes made up of a higher guanosine and cytidine (G+C) content, both of which have a higher ratio of nitrogen to carbon atoms in their molecular makeup. Conversely, organisms that reside in a low-nitrogen environment have genomes made up of a higher fraction of adenosine and thymidine (A+T), both of which have a higher ratio of carbon to nitrogen in their molecular compositions.
Resource-Driven Selection
Given the critical and limiting role carbon and nitrogen play in ecosystems, it isn’t surprising that the Israeli scientists discovered that nutrient availability serves as a driver for purifying selection.
In a nutshell, purifying selection refers to a type of natural selection in which harmful mutations are eliminated from the genomes because they render the organism less fit for the environment. The research team coined the term "resource-driven selection" to describe situations in which nutrient availability provides the dominant contribution to purifying selection.
This process shapes the nucleotide sequences of an organism’s genes so that the genes encode proteins made up of either nitrogen-rich or nitrogen-lean amino acids, depending on the availability of nitrogen in the environment. Along these lines, they discovered that in nitrogen-depleted environments, any mutation that causes an amino acid change in a protein’s primary structure will be "selected against" if the replacement amino acid contains more nitrogen atoms than its predecessor.
The research team also discovered that genes expressed at high levels tended to experience purifying selection to a much greater extent than genes expressed at low levels. This observation makes sense if resource-driven selection is at work.
As part of their investigation, the researchers suspected that the structure of the genetic code, which influences mutational changes, plays a role in resource conservation. Previous research carried out by other investigators has demonstrated that the structure of the genetic code buffers against the harmful effects of single-point and framework mutations. (See the Resources section for articles describing this earlier work.) Based on these earlier studies, the scientists wondered if the structure of the genetic code would also buffer against mutations that would add to the organism’s resource demands.
To fully appreciate their findings and why they pursued this line of inquiry, it is important to have a basic understanding of the genetic code. (For readers who have this understanding, feel free to skip ahead to The Impact of the Genetic Code’s Redundancy on the Effects of Mutations.)
The Genetic Code
The genetic code is a biochemical code comprised of a set of rules that define the information stored in DNA. These rules specify the sequence of amino acids used by the cell’s machinery to synthesize proteins. The genetic code makes it possible for the biochemical apparatus in the cell to convert the information formatted as nucleotide sequences in DNA into information formatted as amino acid sequences in proteins.
Nucleotide triplets or codons represent the fundamental communication units of the genetic code. In other words, the genetic code uses combinations of three nucleotides to signify a particular amino acid in the amino acid sequences used to build proteins.
Sixty-four codons make up the genetic code. Because the genetic code only needs to encode 20 amino acids, some of the codons are redundant. That is, different codons code for the same amino acid. In fact, up to six different codons specify some amino acids. Others are specified by only one codon.
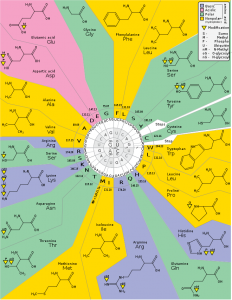
Credit: Wikimedia Commons
Mutations
A mutation refers to any change that takes place in the DNA nucleotide sequence. DNA can experience several different types of mutations. Substitution mutations are one common type. In a substitution mutation, one or more of the nucleotides in the DNA strand is replaced by another nucleotide. For example, an A may be replaced by a G, or a C may be replaced by a T.
This type of substitution changes the codon which is impacted by the mutation. As a consequence, the amino acid specified by that codon may change, leading to an altered chemical and physical profile along the protein chain. If the substituted amino acid possesses dramatically different physicochemical properties from the native amino acid, it can cause the protein to fold improperly. This improper folding can yield a protein with reduced or even lost function. Many mutations harm cellular health because they significantly and negatively impact protein structure and function.
The Impact of the Genetic Code’s Redundancy on the Effects of Mutations
Biochemists have discovered that the redundancy in the genetic code allows the cell to avoid the harmful effects of substitution mutations. For example, six codons encode the amino acid leucine (Leu). If at a particular amino acid position in a protein Leu is encoded by 5' CUU, substitution mutations in the 3' position from U to C, A, or G produce three new codons, 5' CUC, 5' CUA, and 5' CUG, all of which code for Leu. In other words, this mutation does not change the amino acid sequence of the protein chain and, hence, its structure and function. For this scenario, the cell successfully avoids the negative effects of a substitution mutation.
Likewise, a change of C in the 5' position to a U generates a new codon, 5' UUU, that specifies phenylalanine, an amino acid with similar physical and chemical properties to Leu. A change of C to an A or to a G produces codons that code for isoleucine and valine, respectively. These two amino acids also possess chemical and physical properties similar to leucine. It becomes apparent through visual inspection alone that the genetic code appears to be constructed to minimize errors that result from substitution mutations.
The Optimal Genetic Code
As I describe in The Cell’s Design and in articles listed in the Resource section, biochemists have demonstrated that the universal genetic code appears better optimized to withstand the potentially harmful effects of substitution and frameshift mutations than a vast number of other conceivable codes.
Additionally, biochemists have learned that the genetic code’s optimization extends beyond minimizing the effects of mutations. It also displays an optimality that includes its capacity to support overlapping genes and its capacity to harbor overlapping codes. This second feature is vital because in addition to the genetic code, regions of DNA harbor additional overlapping codes that direct the binding of histone proteins, transcription factors, and the machinery that splices genes after they have been transcribed.
The Genetic Code Is Optimized for Resource Conservation
The researchers from Israel added to this impressive list of optimized properties by demonstrating that the genetic code has also been optimized to minimize the excess resource cost that results when one amino acid is replaced with another as a result of a substitution mutation.
Toward this end, the team developed parameters that measure the cost of substituting one amino acid for another based on the number of additional carbon and nitrogen atoms the new amino acid contains compared to the original one. For example, the change of the codon 5' CCA (which specifies proline) to 5' CGA (which specifies arginine) results in an amino acid with 1 additional carbon and 3 additional nitrogen atoms for an N cost of 3 and a C cost of 1.
Using this set of parameters, they calculated that the expected random mutation cost for the genetic code found in nature is 0.44 for carbon, 0.16 for nitrogen, and 0.16 for oxygen.
When researchers compared these parameters for the naturally occurring genetic code with 1 million randomly generated codes, they found that the naturally occurring code has a lower expected random mutation cost than all but 128 random codes. They also discovered that this optimization is independent from the optimization that resists the harmful effects of substitution and framework mutations.
The more scientists learn about the structure of the genetic code found in nature the more remarkable it appears to be. This latest insight not only provides us with a deeper scientific understanding about the nature of biochemical systems, it has profound theological implications.
Codes Come from Intelligent Agents
In The Cell’s Design, I argue from analogy that the mere existence of the genetic code suggests that biochemical systems come from a Mind.
Experience teaches us that it takes intelligent agents to devise codes. The more sophisticated a code, the greater the level of ingenuity required to develop it.
This conclusion gains added support considering the sophistication and the exquisite optimization of the genetic code on multiple fronts. The genetic code found in nature displays a multidimensional optimality that is difficult to explain through evolutionary mechanisms. And, yet, its biochemical properties are precisely what I would expect if life stems from the work of a Mind.
Resources
- The Cell’s Design: How Chemistry Reveals the Creator’s Artistry by Fazale Rana (book)
- “FYI: ID in DNA Deciphering Design in the Genetic Code” by Fazale Rana (article)
- “The Genetic Code: Simply the Best” by Fazale Rana (article)
- “The Optimal Design of the Genetic Code” by Fazale Rana (article)
- “New Insights into Genetic Code Optimization Signal Creator’s Handiwork” by Fazale Rana (article)
- “Origin and Design of the Genetic Code: A One-Two Punch for Creation” by Fazale Rana (article)
- “Deviant by Design, Part 1” by Fazale Rana (article)
- “Deviant by Design, Part 2” by Fazale Rana (article)
- “Mitochondria’s Deviant Genetic Code: Evolution or Creation?” by Fazale Rana (article)
- “Biochemical Synonyms Optimized, Part 1” by Fazale Rana (article)
- “Biochemical Synonyms Optimized, Part 2” by Fazale Rana (article)
Check out more from Reasons to Believe @Reasons.org
Endnotes
- Liat Shenhav and David Zeevi, “Resource Conservation Manifests in the Genetic Code,” Science 370, no. 6517 (November 6, 2020): 683–87, doi:10.1126/science.aaz9642.